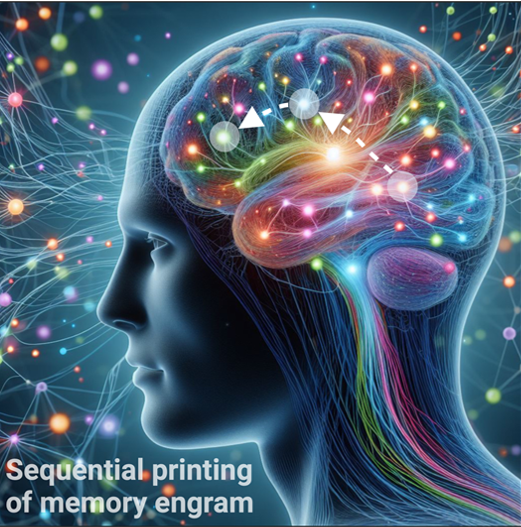
Memories are essential for our daily lives. Memories connect our past, present, and future in a time continuum, enabling us to perform daily tasks in well-executed sequences. Memories shape our personality and make us who we are. Without memories, we would be lost, as in the case of Alzheimer's disease and other memory-related dementia, when connections between brain circuits malfunction and eventually break down, leaving affected individuals with a mental disability in making sense of themselves and the outside world.
Brain circuits are composed of neurons (both excitatory and inhibitory types) and other cell types, such as astrocytes. Neurons communicate with each other by a specific connection made between them called "synapse". Neurons are polarized with two opposite ends: the presynaptic and postsynaptic compartments. In an “activated” neuron, electrical signals in the form of an action potential serves as an information currency that travels along an axon and arrives at the presynaptic terminal compartment called bouton (a tiny protrusion of membrane), where vesicles loaded with neurotransmitters are released into the extracellular space. The presynaptically released neurotransmitters, such as glutamate bind to specific receptors located on the postsynaptic compartment called the spine (a tiny protrusion of membrane) of the other connected neurons. Boutons and spines thus form synaptic connections throughout the brain. In neuron-to-neuron signaling, an electrical signal is converted into a chemical signal, and molecular signaling pathways form anatomical and functional synaptic connections. There are 100 billion neurons in the human brain and each neuron can make up to 1000 connections with other neurons. These connections provide the brain with tremendous computational power, allowing us to do all that we do. Brain is like a very powerful computer, but it’s much more than that, and we are just beginning to understand how the brain works. With constant experiences, synaptic connections are constantly modified; strengthened in some cases and weakened in others. This provides the brain tremendous flexibility to undergo modifications by experience in a process called “synaptic plasticity”. Synaptic connections are strengthened by specific molecules expressed, such as glutamate receptor types, one being the N-methyl-D-aspartate (NMDA) receptor or NMDAR, which upon binding to glutamate in “activated” neurons induces mobilization of intracellular calcium, that acts as a second messenger, to activate molecular signaling in boutons and spines, strengthening synaptic connections; NMDAR thus acts as a "molecular glue" to wire the brain circuits for the formation and preservation of memories. NMDARs are expressed on both presynaptic and postsynaptic neurons and their cooperation is essential for strengthening of synaptic connections. Identifying the different interacting brain circuits and understanding the cellular and molecular mechanisms for learning and memory formation, storage, and retrieval, and how we forget things, and also what goes wrong when memories start to degrade or lost, would help in designing innovative circuit-targeted therapeutics to treat memory dysfunctions as in Alzheimer´s disease. Further, it would guide us to re-engineer brain circuits in precisely targeted manner using advanced and novel molecular and genetic technologies to boost circuit operations for memory enhancement.
Philosophers and scientists have long pondered about the “nature” of memories. More than 2000 years ago, Aristotle (350 BCE) proposed that memory is like a "stamp of a seal," suggesting specificity and physical properties. Fast-forward to the present, Ramon y Cajal (1873) proposed that individual neurons and connections between them are the building blocks of the brain, giving rise to the "Neuron Doctrine.” This reductionist framework led a German Zoologist, Richard Semons (1904), to propose the concept of "memory engram” involving the participation of a set of individual neurons as physical substrates of a memory engram in generating and storing memories. Whether memories were localized or distributed in the brain remained an open question. To address this critical question. Wilder Penfield (1933), an American neurosurgeon, found that about 9% of his patients recalled vivid memories of their past when specific sites in the medial temporal lobe of the patients were electrically stimulated. Penfield concluded that memories are localized in the brain. To identify the location of a particular memory, Karl Lashley (1929), a Canadian zoologist, trained rats to find a hidden reward in a maze. Afterward, Lashley removed pieces of cortex from different areas, but the rat still remembered to find the reward in the maze. This led Lashley to conclude that memories are distributed in the brain. In 1949, Donald Hebb, who had worked with Penfield and Lashley on the nature of memory proposed a pioneering “synaptic plasticity and memory theory” that "neurons that fire together, wire together". Hebb's postulated cell assemblies are strengthened by experience and learning, providing a scientific framework to investigate how memories are formed, stored, and recalled. Over the next several decades, intensive research efforts started to provide evidence that memories are embedded in brain circuits; with evidence that they are both localized and distributed in the brain. However, it remained unclear which neuroanatomical and circuit pathways are used by the brain for forming a memory across the different brain regions and what are the cellular and molecular mechanisms that orchestrate the various aspects of memory functions.
An international team of scientists led by Ikerbasque Professor Dr. Mazahir T. Hasan from the Laboratory of Brain Circuits Therapeutics at the Achucarro Basque Center for Neuroscience have demonstrated in a new publication by Bertocchi and colleagues that fear memory engram is sequentially printed from one specific brain region to the next by “multi-trace systems consolidation” mechanism.
The researchers developed and applied innovative experimental designs and novel genetic technologies with recombinant adeno-associated viruses that can be targeted to specific brain regions and circuits, and they have convincingly demonstrated that the sequential printing of memory engram follow a specific anatomical synaptic pathway from one region to the other and, subsequently, to the next, and so on. First, they used virus-delivered genetic technology and combinatorically blocked synaptic output between interacting brain regions, namely a specific fear center called the basolateral amygdala (BLA), where fear memories are first formed, and then printed to the the medial prefrontal cortex (mPFC) that modulate appropriate response to fear and danger. Second, they genetically removed the NMDARs in a combinatorial manner from these two brain regions and found that postsynaptic BLA NMDARs and presynaptic mPFC NMDARs work together to strengthen synaptic connections. Blocking NMDAR alone from either the BLA or the mPFC did not prevent the formation of memory. However, simultaneous removal of both BLA-NMDAR and mPFC-NMDAR impaired memory formation. The team concluded that the synaptic strengthening requires postsynaptic BLA-NMDAR and presynaptic mPFC-NMDAR. They suggested that experience-activated BLA neurons stimulate mPFC neurons, which in-turn re-stimulate synaptically connected BLA neurons. These reciprocal synaptic interactions possibly operate by secretion of putative diffusible factors between BLA-mPFC circuits, ensuring to exactly print a copy of fear memory engram from BLA to mPFC and subsequently to another brain regions following a similar mechanism. Third, the researchers developed a genetic technology that can selectively “tag fear activated” BLA and mPFC neurons. In the tagged neurons, the researchers expressed a light-activatable microbial protein such as the channelrhodopsin that activates the tagged neurons by blue light stimulation. If BLA prints a memory engram copy onto mPFC, then memory engrams must be present at both locations. After fear experience, photostimulation of the tagged neurons in the BLA alone or mPFC alone was sufficient for fear memory recall. The team thus demonstrated that fear memory is both localized and distributed in the brain and reciprocal connections between BLA are mPFC neurons provide feedforward and feedback control mechanisms in modulating appropriately judged response to fear experiences.
"We have found that the brain circuits act like a printer that makes copies of initially formed memory engram and sequentially print them across the different regions by “multi-trace systems consolidation mechanism," said Prof. Dr. Mazahir T. Hasan. Such a printing mechanism generates engrams across the different brain regions for flexible memory recall along the specific neuroanatomical pathways. The positioning of memory engrams across the different brain circuits modulate behavioral responses, enabling the brain circuits to make conscious decisions to threats and danger. It is conceivable that disruption of excitation-inhibition balance in the BLA-mPFC circuits, as in posttraumatic disorder (PTSD), disables the decision-making process; different BLA-mPFC circuits go into hyper-drive or hypo-drive, leading to inappropriate response in situations that are in fact not threatening. "In the future, it would be necessary to perform circuit targeted neural modulation on identified interacting brain circuits to re-balance excitation-inhibition to restore normal circuit operations as a treatment for PTSD and other emotional dysregulations linked to increased stress, anxiety, and depression, and social susceptibility" concludes Prof. Dr. Mazahir T. Hasan.
Bibliographic reference
Bertocchi et al., “Pre- and postsynaptic N-methyl-D-aspartate receptors are required for sequential printing of fear memory engrams.” iScience. 2023 Sep 25;26(11):108050. doi: 10.1016/j.isci.2023.108050. https://pubmed.ncbi.nlm.nih.gov/37876798/